Materials
- Pythagorean cup (tupperware)
- Colored water
- Container to catch escaping liquid
- Optional: video camera
Procedure
Put the cup into a larger container to ensure no water leaks out onto the demonstration surface. Slowly pour water into the cup until it starts to come out of the bottom. The siphon will ensure the majority of the water in the cup is evacuated into the large container. The video camera may be helpful with this demonstration because it is quite small.
Explanation
The Pythagorean cup, or “greedy” cup is a specially designed cup which will empty its contents once filled to a certain point. As water is poured into the tupperware, it rises up through the central column. Once it reaches the top of the smaller straw inside of the large central column, a siphon will form. Once the siphon in the Pythagorean cup has been “activated”, it will continue to run until all the water in the cup has been emptied in to the container below. It will empty quicker if there is a smaller bubble of air at the bend of the siphon than if there is a larger cavity of air.
Pascal’s Theorem of Communicating Vessels
As water is poured into the cup, it rises and fills the central column. This can be explained by Pascal’s theorem of communicating vessels, which states that when water is poured into two vessels which are connected (so that water can flow between), they will come to equilibrium at the same height. This is because the amount of pressure that water can exert on it’s surrounding is due only to the height of the column of water, and not the shape or width of it. We see this when we look at the equation for the vertical fluid pressure variation:
where is the change in pressure in the vertical column of water,
is the density of the fluid,
is gravitational acceleration (
), and
is the change in height through the column of fluid.
This equation shows that there is no relation between the pressure at a certain point in a column of fluid and the shape of the container that the fluid is in or the area of the column, only the height within the column of fluid at which you are measuring the pressure is relevant.
Once the water level reaches the height of the tube that leads out of the bottom of the tupperware, a siphon is created (even if there is an air bubble at the top of the bend).
Figure 1 (left): Example of a typical siphon, the flow of water is from the reservoir on the left to the one on the right, even though it has to flow up originally
Figure 2 (right): image of the chain example which is a flawed but useful tool in describing the motion of a siphon.
Siphons
A siphon is defined as a tube that is used to bring water up from a reservoir at high elevation against gravity to deposit it in a reservoir at a lower elevation of its own accord (Figure 1). There are two main explanations for how siphons work. At typical atmospheric pressures and temperatures, the prevailing idea for how they work is that gravity pulls down on the water at the lower end of the siphon. As gravity pulls the fluid out of the bottom of the siphon, this causes a low pressure cavity at the top of the siphon (at the bend). When the pressure at the bend is lowered, the weight of the water in the short end of the siphon no longer exerts enough force against the atmospheric pressure pushing the water in the short column up. As a result, in the short column, water is pushed up and over the bend. The water in the higher reservoir then is pulled to the lower reservoir by gravity and the cycle repeats.
It was thought until recently that this was the only mechanism by which siphons worked, but with the discovery that siphons work in a vacuum, it seems that there is another effect as work as well. Atmospheric pressure cannot be a major part of how a siphon works in a vacuum because in a vacuum, air pressure is negligible. This would then not explain why the water was pulled up from the higher reservoir originally. The discovery that siphons worked in a vacuum led to the somewhat flawed chain example that relies on the tensile strength (also known as cohesive forces) within a column of water. Looking at Figure 2, you can see that the chain strung over a pulley will fall down to the lowest point on the right. This is because the length marked “y” is longer than the segment marked “x”. This means that the “y” segment is heavier, and therefore exerts a stronger force than exerted by the right side of the chain. As it falls over to the right side, the chain pulls up the chain from the left, because it is all connected. It is theorized that siphons in a vacuum work in a similar way–that the tensile strength of water is what pulls the water up, working against gravity.
It is worth noting, however, that the chain example isn’t a 1:1 comparison of how the siphon works. In the chain model, the driving force of the shorter chain up and over the pulley is due to the difference in weights of the two sides, whereas with a siphon, the pressure is only due to the relative heights of the two reservoirs. You can even use tubes of such diameters that the weight of the water moving upwards against gravity is greater than the weight of the water moving down in the second column and the siphon will still work (Figure 3). The concept displayed here is not a difference in mass, but a difference in pressure. Another example of how the chain model does not follow reality is that unlike with a chain, most fluids don’t have very great tensile strength–which means that they cannot be “pulled up” on one side as the chain is.
It is likely that both these explanations (the chain model and the atmospheric pressure) work together under most conditions (excepting in a vacuum or with fluids with no tensile strength or surface tension).
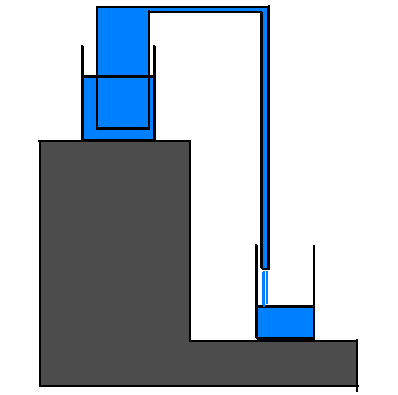
Figure 3: Even when the diameter of the siphon is such that the weight of water on the short column is greater than that of the longer siphon, the siphon will continue to run. This is one of the main differences between the siphon and the chain model mentioned above, which relies on differences in weight to drive motion against gravity.
Written by: Sophia Sholtz